Ferrite: Iron which contains little or no carbon is called ferrite. It is very soft and ductile and is known as alpha iron by the metallurgists. Ferrite is present to some extent in a great range of steels, particularly those low in carbon content, and it is also present, in soft cast iron. Ferrite does not harden when cooled rapidly. Ferrite Microwave Technologies, LLC (FMT) is proud to continue our 35 year legacy of service. Our customers demand the highest performance from their microwave components and systems and we work tirelessly to meet and exceed those requirements.
Q: The analog synth circuit I'm building calls in the schematic for ferrite beads on the power rails, but it doesn't give any further information. What kind of beads should I use?
A: Pick some that will look nice on the board, maybe in a colour that goes with the solder mask. Your local craft supply store should have a good selection of decorative beads, like these glass ones I got in a clearance sale.
Q: But aren't those electrically useless?!?
A: Yes, but so are 'proper' ferrite beads in this context.
Ferrite beads are often misunderstood, and they're included in a lot of SDIY designs by a sort of cargo cult process: people know they want some kind of filtering on power rails, they see ferrite beads used in others' designs, it is a general belief that putting ferrite beads on power rails is the accepted practice, and so they write that into new designs too, which contributes further to the community's perception that that is Just How It's Done. In this article I'm going to go into some detail on what ferrite beads actually are, and why they're useless when we see them on the power rails in most analog synth designs.
Of course ferrite beads are not always useless. They exist as a manufactured product for a reason. I'll discuss below some of the cases where they do serve a useful purpose after all. But in cases where a bead is necessary or appropriate, it will matter what kind of bead to use (because there are different kinds and that makes a difference), and the careful designer who specifies a bead for a good reason, will always give you some details on what kind of bead is needed. Without those details you might as well just use the decorative glass beads from the craft store; and if the details are missing from the design, it's a clue that maybe the designer didn't really understand what they were trying to accomplish when they put that symbol on the schematic.
What ferrite is
The word 'ferrite' has several different meanings in different fields. In metallurgy, it refers to a specific crystalline form of iron metal. But in electronics, it means a ceramic material made of iron oxide combined with certain other metal oxides, commonly used for inductive components. The coils in my Coiler VCF module are wound around ferrite cores.
If you want to build an electronic component with a high inductance, you face a tradeoff. To maximize the energy stored in the magentic field, you need to pass that magnetic field as much as possible through a material with a high ability to accept magnetic field density (a property called permeability). Usually, that means winding a coil around or through a core made of this kind of material. The simplest high-permeability materials are iron and its alloys, which are mostly classified as different kinds of 'steel.'
But iron, like metals in general, conducts electrical current. If you wind a coil on a solid iron or steel core, then a changing current in the coil will induce an eddy current in the core material itself. The core becomes something like a transformer secondary winding, plugged into a short circuit, and that causes power loss and other problems. The eddy currents create magnetic fields of their own, opposing the original field from the main winding, and the opposing fields tend to push the original magnetic field out of the core, harming the intended operation of the component.
So a low-frequency power transformer will often be designed with a steel core that is a stack of flat plates insulated from each other, instead of a single solid piece. The laminated core splits the eddy currents into many small loops instead of a single large loop covering the whole cross section, and many small loops create much less power loss than a single large loop. At somewhat higher frequencies, transformer designers sometimes use powdered iron combined with an insulator that separates the particles. The eddy current loops are limited to the size of the particles instead of the size of the core.
But another approach to avoiding eddy current issues is to use a material with fairly high magnetic permeability (even if not quite as high as a metallic alloy), but high resistance to electricity. That is the purpose of ferrite: it is a substance that accepts a lot of magnetism, but basically an insulator to electrical current. Ferrite is magnetic iron oxide with some alloying elements. The mineral magnetite can be thought of as a primitive ferrite, though artificial substances made for the purpose are much more effective.
Ferrite achieves high permeability with low conductivity by containing iron nuclei in a crystal structure that allows them to be magnetic but does not have the conduction band of electron states that is present in metallic forms. Without the conduction band there's no easy way for a current to pass through the crystal. A somewhat oversimplified intuition is that the oxygen atoms in the crystal are acting as insulators between the iron atoms.
Ferrites can be divided into 'hard ferrites' and 'soft ferrites,' formulated with different alloying elements. These words do not refer to mechanical hardness but to the oxides' magnetic properties, by analogy to 'hard' and 'soft' magnetic iron. In the case of metallic iron, the words hard and soft do correlate with mechanical hardness even though it's not the main point. Hard ferrites can be made into permanent magnets and are typically used for stuff like sticking papers to refrigerators, where it's not necessary to use the more powerful and expensive rare-earth magnets. Soft ferrites are the ones we usually care about in electronics: they do not accept permanent magnetization, but have the necessary high permeability to create high-inductance magnetic components. Ferrite beads for interference suppression are made out of soft ferrite; the tiny ferrite cores in old-fashioned computer core memory were made of hard ferrite.
The two faces of soft ferrite
The Fair-Rite Products Corporation has a downloadable catalogue containing a lot of useful technical information about ferrite materials and the components made from them. This diagram from page 12 representing the complex permeability of '61 Material' as it varies with frequency, illustrates the two basic operating modes of soft ferrite material.
At relatively low frequencies, the high permeability of the ferrite allows it to soak up a large magnetic field, giving inductive electrical behaviour. The electrical resistance of the ferrite is also high, so little or no current flows through the ferrite core itself, and there is little power loss. But as the frequency increases, the inductive reactance increases too because that is the nature of inductive reactance, while the resistance of the core remains basically unchanged. At sufficiently high frequencies, the resistance of the core is going to become small relative to the prevailing impedance of eddy currents, and at that point the current flowing through the resistive core becomes important. The component stops behaving as a pure inductor and becomes quite lossy. So there are two kinds of behaviour, depending on frequency: at lower frequencies it's a high-value, low-loss inductor, and at higher frequencies it's lossy, dissipating most of the input power.
Both kinds of behaviour are useful. If we're building a broadband transformer, or a simple inductor for something like a communications radio, then we want high inductance at a few megahertz, and low loss. We get that in the portion of the chart where µ's (indirectly associated with inductance) is dominant. If we're building a component that is meant to filter out noise in the VHF to UHF range (hundreds of MHz), then we want it to dissipate the power from the noise, and that happens at higher frequencies when the other curve, labelled µ's, starts to increase seriously.
The Fair-Rite catalogue describes 61 Material in particular as appropriate for inductive applications below 25MHz and noise suppression above 200MHz, and that's what we can see in the chart. Most of their other ferrite materials are only really intended to be used in one of these modes or the other, but a similar two-phase pattern of behaviour (not always so cleanly divided) is visible in the similar charts for all the different materials in the catalogue.
Ferrite beads
A current through a straight piece of wire creates a magnetic field in a ring surrounding the wire. When we build an inductor we normally form the wire into a coil, allowing the field from each turn to reinforce that of all the other turns; the inductance scales with the square of the number of turns. To boost the inductance further, we might put a ferrite core in the middle where the field can pass through it; the ferrite material is able to support much more magnetic flux than empty space. But the very simplest way to use a ferrite core might be to just have a plain wire and put ferrite where the magnetic field naturally occurs: that is, slip a ferrite bead over the wire. And one advantage to doing that is that we can do it even in places it would be inconvenient to put an entire, more complicated, inductor assembly. We can wrap a ferrite bead around an entire multi-conductor cable, like this USB cable with a built-in plastic-encapsulated ferrite interference filter.
The signal conductors in that cable are twisted pair transmission lines and they are to some extent self-shielding: the differential signals carried on them do not create an external magnetic field that will interact significantly with the ferrite. But if there should be a common-mode signal propagating on the cable as a whole, for instance if this cable is used in the vicinity of a strong radio transmitter, then that signal will create a field in the ferrite and if the frequency is high enough, the ferrite will dissipate its power. A similar effect occurs in both the transmitting and receiving direction: this cable will both pick up, and generate, less radio interference than would a cable without a ferrite bead.
Ferrite beads are used on individual conductors when there's a concern that there may be RF floating around and none of that is wanted on the particular conductor in question. For example, if you're building a single-board computer you might have a CPU with a clock frequency in the hundreds of megahertz. It's quite likely that some of that frequency will be coupled into the power rails, and other parts of the system may be sensitive to it. The decoupling capacitors ought to eat most of the clock exposure to the power supply, but designers may put beads on the power lines leading into the CPU to absorb any of the radio-frequency clock that may still be present, and also on lower-frequency signal lines elsewhere to prevent it from leaking in.
To be perfectly honest (and as implied by some of the discussion in the Fair-Rite catalogue), a big part of the reason for using ferrite beads is just to satisfy government regulators. The certification labs will point antennas at your product. If the product radiates too much radio-frequency energy, you fail; and if they tickle it with a radio transmitter and the product stops working, you fail again. So commercial designers will add ferrite beads, designed to operate in their lossy region, until it seems clear that there won't be significant radio frequency energy coming into or out of the product.
Here's another chart from the Fair-Rite catalogue, page 146; the article 'How to Choose Ferrite Cores for EMI Suppression' starting on that page is a useful resource. The plot shows the impedance of a typical ferrite bead, with the magnitudes of the resistive (R), inductive (XL), and total (Z) components. Note that the total is not the simple arithmetic sum because the other two are at right angles to each other.
As predicted by the theory of ferrite behaviour above, the impedance starts near zero (inductors are like plain wires at low frequencies), then grows with frequency while being mostly inductive. Around 20MHz (in this case) the inductive reactance starts to fall away, but resistance becomes a bigger issue, and the beaded wire behaves more like a resistor, up to a peak in the hundreds of megahertz. The following equivalent circuit produces similar behaviour and may make clear what's going on.
Here are similar impedance curves for the equivalent circuit. They are not identical to the chart from the catalogue; in particular, the peak of inductive reactance for the real-life bead is not as high in relation to resistance as it is with the equivalent circuit because of the frequency dependence of the resistance, which is not modelled here. Simulating that effect would require a more complicated equivalent circuit and would not really make things any clearer. I just chose values for the equivalent components to be reasonably plausible and give similarly-shaped curves to the real bead.
Dirty power in analog SDIY
Synthesizers generate audible signals, and when people complain about noise in synth circuits, they are complaining about audible noise. That means noise in the range 20Hz to 20kHz. How many forum threads have you read in which people griped about 'bleed' in VCAs - because they could hear the input signal coming through to the output even when the VCA was supposed to be turned off - only to eventually discover that the signal was actually passing through the power distribution system? That and the audible hiss often blamed on the presence of digital modules are both caused by audio-frequency signals carried on the power rails.
So people put a lot of effort into reducing the transmission of audio frequencies through the power system of a modular synthesizer. (Similar considerations apply also to non-modular synthesizers, which still have internal divisions into different sections.) One thing to do is to use a power supply with a good voltage regulator, capable of keeping its output at a fixed voltage. Voltage regulators in this mode are essentially working as audio amplifiers for the signal frequencies involved, generating audio-frequency power of their own to counteract whatever is being imposed on the power line by other parts of the system. But the voltage regulator is only capable of regulating the voltage at one point, its output terminal or at best the location of its remote-sensing tap.
Given a single point in the circuit considered to be constant-voltage with minimal audio-frequency interference, the next step is to keep the impedance as low as possible on any power lines from there to the modules that happen to be shared among more than one module. If one module is putting an audio-frequency signal onto the bus at one point, and another module is connected to the bus in such a way as to share some of the wire between there and the point of purest power, then the audio signal dropped across that shared wire will result in a voltage fluctuation seen by the second module, no matter how pure the power was at the source.
Impedances in shared connections lead to cross-talk between modules, both of intelligible signals (perceived as 'bleed') and generic hissing sounds (perceived as 'noise'). High-end bus-bar power systems for modular synths aim to reduce those shared impedances to be as small as possible, both by using large conductors with low resistance, and by routing the conductors all to a common power point to reduce the shared wiring. On the other end of the scale, the 'flying bus' cables sometimes recommended for starter systems place a lot of thin, shared wires, with relatively high impedance, between modules and the power supply. Those systems are more likely to have module-to-module interference problems.
The third thing people do to try to reduce the dirty-power problem is build filters between the modules and the power system. Whereas low impedance on shared power connections is desirable, for the power feed to an individual module that is not shared with others you want the impedance at audio frequencies to be as high as possible, the better to block audio-frequency power from other modules coming in or from this module going out. The filter still needs to pass the DC power actually required by the module.
That is where ferrite beads come in. Designers know that ferrite beads can pass DC well - after all, there is a wire right through the bead, and at DC the magnetic effects make no electrical difference - and the beads become resistive at higher frequencies. That's what we need from a power filter, right? So they specify ferrite beads on the power rails.

Maybe you have noticed the problem already.
Why the beads are useless
Ferrite beads are designed to block conducted interference at radio frequencies. Radio frequencies are not what the typical analog SDIY circuit needs to be protected from! The 'noise' and 'bleed' problems for which people want to deploy beads, are happening at audio frequencies, where the beads do nothing.
My frequency-impedance plots above start at 1MHz, which is 50 times the highest audible frequency. Audio frequencies are far off to the left of the diagram, where the impedance is essentially zero. The Fair-Rite Products Corporation doesn't bother to specify any of its EMI-suppression beads at frequencies below 1MHz, and most are designed to run at higher frequences, into the tens or hundreds of MHz. Ferrite bead products from other manufacturers have similar specifications.
By all means there are ferrite products designed to run at lower frequences - many of them are in the Fair-Rite catalogue - but those are operating in the inductive range of ferrite behaviour, they use far more turns to produce a meaningful inductance instead of just running a single wire once through a bead, and even then they are usually meant for higher frequencies than audio. The EPCOS ferrite-core inductors in my Coiler VCF are being pushed to the lower limit of their frequency range. Inductors and inductive components like transformers designed for audio often use laminated steel cores instead. There just aren't any ferrite materials that run in the lossy mode at audio frequencies.
Looking at the equivalent circuit again, consider the three components. The idealized 220Ω resistance will be 220Ω regardless of frequency. In the audio range, we get the inductive reactance of the inductive equivalent component by multiplying kilohertz by one microhenry, giving milliohms. The capacitive reactance is given by kilohertz times picofarads, giving nanosiemens, which invert to gigaohms. The low inductive reactance shorts out the resistance and capacitive reactance and the overall behaviour is basically the same as just putting a plain wire on the board, or running the wire through one of my decorative glass beads. At audio, the ferrite bead has no effect.
So could it ever make sense to use a ferrite bead in a synthesizer? Yes, but only in limited circumstances, only with thought and testing, and only if we're also going to do other things that SDIY designers often skip.
I have carefully said 'analog SDIY' in most of this article. There are certainly synthesizer circuits that aren't analog. If you have a microcontroller in your design, it may have a clock in the tens of MHz range, and you might be worried about signals of that frequency getting from the module to the power bus, or coming in through the power bus. I would normally expect bypass capacitors to be the first line of defence there, but it's possible that on careful analysis one could find that a ferrite bead makes sense. Many ferrite beads work well in the tens of MHz range. Of course then it would be necessary to specify which bead, and especially which ferrite material, to suit the range of frequencies that are of concern.
But it's important to distinguish that kind of thing - interference to and from digital circuits at the radio frequencies they use internally - from the audio-frequency 'digital noise' synth users love to complain about. Audio-frequency stuff, regardless of whether it is called 'digital,' goes right through ferrite beads as if they were just plain wires.
There is some possibility that a ferrite bead might be used to calm down parasitic oscillation on a high-performance op amp, especially if it's an amplifier really built for higher-than-audio frequencies being pressed into audio service. It's usually more popular to use a capacitor in parallel with the feedback loop, but an inductance in series - provided by a ferrite bead on the amplifier output - could have a similar effect. I think the capacitor would usually be easier to design.
The other way ferrite beads could become relevant to synthesizers is when it comes to radiated noise, again both transmitted and received. An analog synth circuit is very unlikely to generate any signals above about a hundred kilohertz, which is still too low for a ferrite bead to block them. But a digital circuit that puts its clock frequency onto the power bus may turn the bus into an antenna, radiating a signal that will cause the system to fail regulatory tests even if there is no audible effect on other modules.
And if a modular synth is being used in an environment of high radiation from other things (maybe on a stage where there are a lot of wireless mics in use, or in some scenarios involving ham radio transmission), it's possible to imagine that the power system might pick up enough radio waves that they could screw up a module that was sensitive to radio frequencies. In the extreme case, components in an analog synth circuit could demodulate the radio signals back into audio, resulting in wireless 'bleed,' though that would probably require radiation levels so high as to overwhelm ferrite-bead filters anyway.
Ferrite beads might become relevant in a synthesizer in those kinds of cases, when there is a specific radiation-related interference problem. But it's pretty much for nothing if you aren't also using a shielded enclosure. The average wooden or plastic Eurorack enclosure is transparent to radio waves. Block them where the power cable attaches to the module, and they will just be picked up again on the other side of the filter. You need an all-metal or at least foil-lined case before there will be much point thinking about ferrite beads for radiated interference concerns, and you need to be careful about proper handling of the shield connections on patch cables. Just forget it in an unshielded banana-jack synth format.
So I've gone through what ferrite is, how it behaves in electronic components, and the use and misuse of ferrite beads. Although there are cases where ferrite beads could be useful in a synth design, by far the most common ferrite bead application that synth DIY hobbyists ask about is the idea of putting them on the DC power inputs to an analog module as a prophylactic against 'noise.' There's often confusion about which specific model of beads to use in that power filtering application, and the fact is, if you're concerned about audio frequencies then you might as well just use decorative glass beads for all the good they'll do.
Previous entry: Designing for adjustment || Next entry: Toronto Pedal and Synth Expo
These tests and experiments were performed by Ian Jackson VK3BUF in the QRM Guru test lab.
Much has been said about the importance of applying ferrite clamps, rings and beads to radios and other domestic products to fight QRM.
Articles on the correct placement of ferrite noise suppressors are common, but little has been written about the different options and where to buy them. In Australia, there are only a limited number of suppliers that carry stock. Ferrite size, shape and cost varies significantly. The information provided can be minimal or non-existent. Part numbers for ferrites listed in international catalogues are not generally available in Australia and buying these can entail long lead times and high freight costs.
Often we don’t really know what we’re getting and how effective they will work for us when they finally arrive. From this perspective, buying and using ferrite filters seems to have more in common with black magic than the application of radio science.
- How do I know if the ferrites I purchased are good, bad or totally ineffective?
- Do I get what I pay for? Are expensive ferrites much better than cheap ones?
- How can I tell if one ferrite is enough? Are 2 or 3 together really worthwhile?
- What are the advantages of clamps over beads and rings?
- Are big and heavy ferrites better than lightweight and small ones?
- How far up the radio spectrum are these things going to work for me?
Ferrites are a type of ceramic made from iron and other oxides and come moulded into different shapes. The material combination is called a ‘mix’.
The characteristics of these mixes determine where and how they should be used. When a wire passes through or near ferrite materials, it effectively adds resistance to that wire at radio frequencies, but this resistance effect varies with the frequency applied to the wire.
Every ferrite has its own characteristic impedance curve allowing it to absorb unwanted RF currents before reaching your receiver or appliance.
Unfortunately, you can’t tell what that working curve is going to be just by looking at it.
For this experiment, we purchased sample ferrites from the Australian retailers Jaycar and Altronics. We compared these with samples of similar size from the QRM Guru ferrite kits, then further compared all of these with some cheap ‘no-name’ ferrites purchased from eBay.
Testing methodology is important. We used a spectrum analyser with a tracking generator. The spectrum analyser shows gain or loss of radio frequencies between any two points on the radio spectrum. Our unit has capability to scan the radio spectrum from 10KHz to 1.5 GHz, but in this trial, we profiled these ferrite devices between 100KHz and 450MHz.
The tracking generator creates a small signal that regularly sweeps between two frequencies we are monitoring at a very controlled level. We are then able to couple from the tracking generator to the spectrum analyser via a small brass rod, which will become our test wire. First we ‘normalise’ to compensate for any stray capacitance and inductance around our test area. A flat yellow line represents zero dB. This line becomes our reference point before filtering is added. When any unknown ferrite is added to the test conductor, we measure a clear plot showing the unique characteristics of that item.
With this arrangement we examined a graphic plot of each sample, then recorded five unique values which identify its effectiveness. We looked at :
- (A) The lowest frequency where the item drops below the -3dB (half-signal) point.
- (B) The highest frequency where the curve crosses the -3dB point
- (C) The frequency (MHz) where the greatest attenuation takes place
- (D) The maximum degree of attenuation (peak –dB) that takes place.
- (E) The weight of each ferrite item. (in grams)
Not all characteristics are being tested here
It should be noted that this article is focused on using ferrites for noise reduction only. In this role the energy being absorbed is not great. Where ferrites are used in high-current environments, such as in a transmitter balun, there will be an RF power threshold where they can no longer effectively absorb energy and their characteristics will begin to distort.
The overheating and saturation effects of high current applications are not a part of this study.
Study Results
The table below contains the raw results of our assessment, grouped in order of supplier, then size. The figures can be difficult to digest in this form, but we can take away some very important findings from this test data.
Branding vs Price
The first significant observation is that they all worked. Regardless of the source, none of the samples tested were fake or defective. They were all capable of suppressing radio frequencies to a greater or lesser degree. This is reassuring, as you can never prove authenticity just by looking at a ferrite.
From these samples, we can surmise that regardless of their source, all of these clamp-on ferrites will provide at least some degree of effectiveness in the shack.
Ferrite Ring
Ferrite Mixture Type
The next observation (with the exception of the Altronics Iron Core ring) is that all of these materials are composed from a similar mix of ferrite material. The absorption curves were all a reasonable match to Mix 43. This indicates that their application is appropriate for HF and low VHF frequencies. (The Altronics ring L4534A was purchased with the rest of the ferrites, but it does not qualify as a ferrite device. It is a powdered iron object with different characteristics. We left it in the test for contrast and will provide separate comment on these devices.)
Size vs weight correlation of ferrite clamps
Clamp-on ferrite devices come in a variety of different physical sizes and shapes. It is reasonable to think that bigger ferrites work better than smaller ones, but is this actually the case? We sorted our samples by weight and plotted this chart to see how closely the degree of absorption of RF energy followed the physical weight of each sample.
The answer appears to be”partially yes”. Generally, heavier ferrites will out-perform lighter versions, but there were a couple of exceptions that did very well.
The Altronics solid ring L4534A (A3) outperformed clamps of similar weight, as did the JaycarLF1294 (J2). Both are circled in red in the chart above.
There is a correlation between ferrite effectiveness and wall thickness. Both circled examples had a relatively small internal wire opening, which made for a thicker clamp wall. This equates to a higher density of ferrite material around the single conductor, giving superior performance.
Conversely, sample E1 from eBay was a physically large clamp, but it catered for a thick 12mm cable. This thinner wall thickness reduced effectiveness, as seen by the data point circled in yellow on the chart above.
This effect is not as bad as it first seems, so don’t necessarily shop only for thick-walled clamps. Examine some of the following sections in this article which explore the most effective ways to use ferrite clamps.
The final comment on the size of clamps was the observation that the very small clamp-on ferrites don’t have sufficient mass for good performance. The smallest of the eBay clamps (E5) didn’t even reach -3dB. Unless a friend gives you a lot of them for nothing, there is a lot to be said for aiming straight for medium and large clamps, or the results could be disappointing.
Are solid ferrite rings better than split rings?
This turned out to be an easy question to answer. Two rings of similar weight, hole-size and ferrite mix were compared. One was solid and the other was split.
The results were almost identical when tested. This tells us that shopping for solid cores have no real advantage over split cores. However, the split ferrites can be applied to cables without having to remove connector plugs and this makes them a more practical purchase.
What’s the best way to use ferrites?
Ferrite Core
An important question to answer is “what is the optimum configuration for the application of ferrite clamps“. It’s easy to imagine that two clamps are better than one, but how much better?
For this exercise we focused our attention on the QRM Guru clamps (Q2) designed for 8mm wire.

Compare the following screen captures and images of one and two clamps:
Unsurprisingly, doubling up on the clamps gives an additional 3dB of RF absorption. At 55 MHz the peak absorption jumped from -4.40 dB to -7.54 dB. This tells us that if one clamp on its own does not quite do the job, then add another for improved performance. Be aware that to achieve a further 3dB, you must double again from two to four units.
Next, we explore the effectiveness of looping a wire through a clamp more than once. This can only work where there is sufficient slack in the cable, and where the hole in the core is large enough to accept additional turns.
Here is what happens:
This is a very interesting result showing that the single extra turn through the core increases absorption from -4.4dB to a huge -12.41 dB. This 8 dB increase makes a single clamp provide the same effectiveness of around six such clamps on the same wire.
Want to see some case studies covering off the use of ferrites? Click on the links below
Why adding turns makes ferrites much more effective
This effect is not really a mystery when we break down what’s happening. When we add ferrite to a wire, we are effectively adding series resistance to that wire, but this resistance effect varies with frequency. At steady DC, the ferrites have no effect, but as an AC signal is applied a resistance is developed within the wire surrounded by the ferrite material. The higher the frequency, the greater the increased resistance. This resistance is also affected by the type of ferrite material, its volume, and its distance from the wire concerned.
When we increase the windings through a core, we begin to invoke conventional transformer theory as well. As happens in power transformer calculations, the impedance ratio is always the square of the turns ratio. So increasing turns through the core by x2 lifts its impedance (and attenuation effect) by x4. A x4 increase equates to a 6dB increase. In our example above we had a larger 8dB change. This was because on the first turn, where the wire just passed through a clamp, it was not in contact with the ends of the clamp. Hence the first complete loop through the core provides more benefit than just one turn.
Earlier we observed that thin wall clamps had poorer performance than thick wall clamps. However, if the reduced wall thickness then allows multiple turns through the clamp, it will more than make up for the initial poor response afforded by a single wire passage.
The big takeaway from this observation is to insert the maximum possible turns through any ferrite clamp. This is always going to be cheaper and more compact than adding extra clamps to the same wire. If more suppression is needed, perform both actions.
Ferrite clamps vs ferrite rings
Initial test results of ferrite rings showed poor performance compared to clamps when a single wire was passed through a ring. This does not mean that ferrite rings are a poor choice. Ferrite rings are a convenient way for a large cable to achieve multiple turns and high exposure to the ferrite material.
In the example below we can see the effects of a single wire passing through a large ferrite ring both once and then in another experiment using four complete turns.
Certainly, there is ample room for adding even more windings, but the rapid escalation of HF absorption becomes very apparent from just four turns.
Some unpredictable dips and distortions begin to appear in the plot at VHF and UHF frequencies. This is mostly due to increased stray capacitances and resonance effects of large rings interacting with the wiring.
Ferrite rings become very practical choices where cables are thick and a lot of suppression is needed. There is a lot of ferrite volume in the larger rings, permitting a lot of energy absorption.
Stacking rings before windings are applied are another excellent way of increasing that volume and overall effectiveness.
One precautionary comment: A high turns-ratio on a ferrite ring also generates high circuit voltages if there is significant energy being dissipated. This effect can be cancelled out without affecting absorption if the ring has balanced windings between two sides of the ring
How important is it to get the right Mix?
As previously described, ferrite products are created with different mixture profiles. These different mixes absorb RF energy in different parts of the spectrum. Thus far we have examined a range of common ferrite clamps and rings which profile as ‘Mix 43’, which is a common, general purpose filter. However some operators are keen to obtain maximum effect on lower HF bands and in this area Mix 31 is reputed to be better. This mix is generally harder to get and more expensive, so it would be nice to know if it is worth the effort.
In this final experiment we purchased some genuine Fair Rite samples graded as Mix 31. This was a very close physical match in size, shape and weight to the Jaycar LF1290 with its Mix 43 profile. A good physical match was important for realistic comparisons at HF frequencies.
The results confirm that the Mix 31 ferrites can absorb RF at lower frequencies than the more readily available Mix 43 composition. With the single passage of a conductor at 3MHz there was a measurable absorption difference of 2.4 dB. These two curves quickly begin to converge with a rise in frequency. At 30 MHz the difference was a scant 0.8 dB. By 150 MHz the two lines follow a near-identical path.
On the 80 metre HF band, the Fair Rite ferrite with its lower mix value delivers superior results, but the conventional Jaycar sample is not far behind. As seen with our previous measurements, absorption effects will compound where multiple turns of conductors are applied.

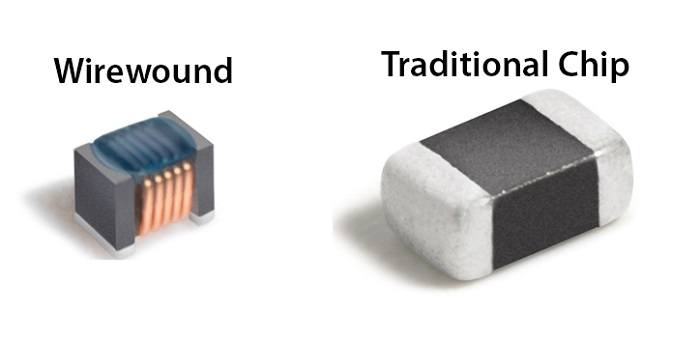
In practice, a single passage of cable through either type of clamp would barely create any discernible difference in Common Mode noise. Multiple turns are needed. The success of any good filtering exercise will therefore be governed by the number of turns that may be applied to a clamp, individually or as part of a chain of ferrite devices.
Summary
Ferrites have an important role to play in suppressing RF noise. These experiments and tests have illustrated the underlying science behind the practical application of ferrites in your desire to eliminate QRM. Some of the mystery surrounding ferrites have also be addressed. Much has been written on this topic and many detailed documents are available for those who wish to dig a little deeper. For most operators, this article will provide sufficient information to get started.
All ferrite devices exhibit a curve and a frequency where they are at their most effective. Just because the peak frequency is not where you wish to operate, doesn’t mean that the device won’t be fit-for-purpose in other parts of the spectrum. It’s all about having sufficient filtering to do the job at hand without blowing the budget.
As always, it is good to share experiences and achievements with others, so if you have a success story in the elimination of interference with ferrite clamps and rings, please forward notes to feedback@qrm.guru
Thanks Ian VK3BUF for a practical insight into this complex topic. And thanks also to Leigh VK5KLT for conducting a peer review.
Want to see some case studies covering the use of ferrites? Click on the links below
Ian VK3BUF: “The Effectiveness of Ferrites”
Josh KI6NAZ: “For most of us, RFI is around us all the time“.
Peter VK3YE:“The best thing you can do for your ham station“
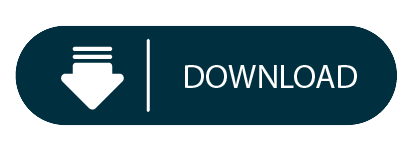